Corona solar: diferència entre les revisions
→Erupcions: Retocs |
Cap resum de modificació |
||
Línia 138: | Línia 138: | ||
{{AP|Ejecció de massa coronal}} |
{{AP|Ejecció de massa coronal}} |
||
Sovint, acompanyades de grans erupcions solars i prominències, hi ha ejeccions de massa coronal. Es tracta d'emissions enormes de material coronal i camp magnètic que viatgen cap a l'exterior des del Sol a més d'un milió de quilòmetres per hora, que contenen aproximadament 10 vegades l'energia de la erupció solar o la prominència que les acompanya. Algunes ejeccions de massa coronal més grans poden propulsar centenars de milions de tones de material a l'[[espai interplanetari]] a aproximadament 1,5 milions de quilòmetres per hora.{{Cal citació|data=febrer 2022}} |
Sovint, acompanyades de grans erupcions solars i prominències, hi ha ejeccions de massa coronal. Es tracta d'emissions enormes de material coronal i camp magnètic que viatgen cap a l'exterior des del Sol a més d'un milió de quilòmetres per hora, que contenen aproximadament 10 vegades l'energia de la erupció solar o la prominència que les acompanya. Algunes ejeccions de massa coronal més grans poden propulsar centenars de milions de tones de material a l'[[espai interplanetari]] a aproximadament 1,5 milions de quilòmetres per hora.{{Cal citació|data=febrer 2022}} |
||
<!-- |
|||
==Stellar coronae== |
|||
Coronal stars are ubiquitous among the stars in the cool half of the [[Hertzsprung–Russell diagram]].<ref name=Gudel>{{cite journal |author=Güdel M |title=X-ray astronomy of stellar coronae |journal=The Astronomy and Astrophysics Review |year=2004 |volume=12 |issue=2–3 |pages=71–237 |doi=10.1007/s00159-004-0023-2 |url=http://astronomy.sci.ege.edu.tr/~rpekunlu/GBDG/papers/XRayfromStellarCoronae.pdf |arxiv=astro-ph/0406661 |bibcode=2004A&ARv..12...71G |s2cid=119509015 |archive-url=https://web.archive.org/web/20110811230835/http://astronomy.sci.ege.edu.tr/~rpekunlu/GBDG/papers/XRayfromStellarCoronae.pdf |archive-date=2011-08-11 }}</ref> These coronae can be detected using [[X-ray telescope]]s. Some stellar coronae, particularly in young stars, are much more luminous than the Sun's. For example, [[FK Comae Berenices]] is the prototype for the FK Com class of [[variable star]]. These are giants of spectral types G and K with an unusually rapid rotation and signs of extreme activity. Their X-ray coronae are among the most luminous (''L''<sub>x</sub> ≥ 10<sup>32</sup> erg·s<sup>−1</sup> or 10<sup>25</sup>W) and the hottest known with dominant temperatures up to 40 MK.<ref name=Gudel/> |
|||
The astronomical observations planned with the [[Einstein Observatory]] by Giuseppe Vaiana and his group<ref name=Vaianaetal81>{{cite journal |doi= 10.1086/158797 |last=Vaiana |first= G.S. |title= Results from an extensive Einstein stellar survey |journal= The Astrophysical Journal|year=1981 |volume=245 |page=163 |bibcode=1981ApJ...245..163V|display-authors=etal}}</ref> showed that F-, G-, K- and M-stars have chromospheres and often coronae much like the Sun. |
|||
The ''O-B stars'', which do not have surface convection zones, have a strong X-ray emission. However these stars do not have coronae, but the outer stellar envelopes emit this radiation during shocks due to thermal instabilities in rapidly moving gas blobs. |
|||
Also A-stars do not have convection zones but they do not emit at the UV and X-ray wavelengths. Thus they appear to have neither chromospheres nor coronae. |
|||
==Physics of the corona== |
|||
[[File:171879main LimbFlareJan12 lg.jpg|thumb|left|This image, taken by [[Hinode (satellite)|Hinode]] on 12 January 2007, reveals the filamentary nature of the corona.]] |
|||
The matter in the external part of the solar atmosphere is in the state of [[plasma (physics)|plasma]], at very high temperature (a few million kelvin) and at very low density (of the order of 10<sup>15</sup> particles/m<sup>3</sup>). |
|||
According to the definition of plasma, it is a quasi-neutral ensemble of particles which exhibits a collective behaviour. |
|||
The composition is similar to that in the Sun's interior, mainly hydrogen, but with much greater ionization of its heavier elements than that found in the photosphere. Heavier metals, such as iron, are partially ionized and have lost most of the external electrons. The ionization state of a chemical element depends strictly on the temperature and is regulated by the [[Saha equation]] in the lowest atmosphere, but by collisional equilibrium in the optically thin corona. Historically, the presence of the spectral lines emitted from highly ionized states of iron allowed determination of the high temperature of the coronal plasma, revealing that the corona is much hotter than the internal layers of the chromosphere. |
|||
The corona behaves like a gas which is very hot but very light at the same time: the pressure in the corona is usually only 0.1 to 0.6 Pa in active regions, while on the Earth the atmospheric pressure is about 100 kPa, approximately a million times higher than on the solar surface. However it is not properly a gas, because it is made of charged particles, basically protons and electrons, moving at different velocities. Supposing that they have the same kinetic energy on average |
|||
(for the [[equipartition theorem]]), electrons have a mass roughly {{gaps|1|800}} times smaller than protons, therefore they acquire more velocity. Metal ions are always slower. This fact has relevant physical consequences either on radiative processes (that are very different from the photospheric radiative processes), or on thermal conduction. |
|||
Furthermore, the presence of electric charges induces the generation of electric currents and high magnetic fields. |
|||
Magnetohydrodynamic waves (MHD waves) can also propagate in this plasma,<ref name="Jeffrey">{{cite book |
|||
|last = Jeffrey |
|||
|first = Alan |
|||
|year =1969 |
|||
|title = Magneto-hydrodynamics |
|||
|publisher = UNIVERSITY MATHEMATICAL TEXTS |
|||
}}</ref> even though it is still not clear how they can be transmitted or generated in the corona. |
|||
===Radiation=== |
|||
{{see also|Coronal radiative losses}} |
|||
Coronal plasma is optically thin and therefore transparent to the [[electromagnetic radiation]] that it emits and to that coming from lower layers. The plasma is very rarefied and the [[photon]] [[mean free path]] overcomes by far all the other length-scales, including the typical sizes of common coronal features.{{citation needed|date=January 2022}} |
|||
Electromagnetic radiation from the corona has been identified coming from three main sources, located in the same volume of space: |
|||
* The K-corona (K for ''kontinuierlich'', "continuous" in German) is created by sunlight [[Thomson scattering]] off free [[electron]]s; [[doppler broadening]] of the reflected photospheric [[absorption line]]s spreads them so greatly as to completely obscure them, giving the spectral appearance of a continuum with no absorption lines. |
|||
* The F-corona (F for [[Joseph von Fraunhofer|Fraunhofer]]) is created by sunlight bouncing off dust particles, and is observable because its light contains the Fraunhofer absorption lines that are seen in raw sunlight; the F-corona extends to very high [[elongation (astronomy)|elongation]] angles from the Sun, where it is called the [[zodiacal light]]. |
|||
* The E-corona (E for emission) is due to spectral emission lines produced by ions that are present in the coronal plasma; it may be observed in broad or [[forbidden line|forbidden]] or hot [[spectral line|spectral emission lines]] and is the main source of information about the corona's composition.<ref name="Corfield">{{cite book |
|||
|last=Corfield |
|||
|first=Richard |
|||
|year=2007 |
|||
|title=Lives of the Planets |
|||
|publisher=Basic Books |
|||
|isbn=978-0-465-01403-3 |
|||
|url-access=registration |
|||
|url=https://archive.org/details/livesofplanetsna00corf |
|||
}} |
|||
</ref> |
|||
===Thermal conduction=== |
|||
[[File:STEREO-A first images.jpg|thumb|A mosaic of the extreme ultraviolet images taken from [[STEREO]] on December 4, 2006. These false color images show the Sun's atmospheres at a range of different temperatures. Clockwise from top left: 1 million degrees C (171 Å—blue), 1.5 million °C ({{gaps|195|Å—green}}), {{gaps|60|000}}–{{gaps|80|000|°C}} (304 Å—red), and 2.5 million °C (286 Å—yellow).]] |
|||
[[File:STEREO-A first images slow anim.gif|thumb|[[STEREO]] – First images as a slow animation]] |
|||
In the corona [[thermal conduction]] occurs from the external hotter atmosphere towards the inner cooler layers. Responsible for the diffusion process of the heat are the electrons, which are much lighter than ions and move faster, as explained above. |
|||
When there is a magnetic field the [[thermal conductivity]] of the plasma becomes higher in the direction which is parallel to the field lines rather than in the perpendicular direction.<ref name="Spitzer">{{cite book |
|||
|last=Spitzer |
|||
|first= L. |
|||
|year=1962 |
|||
|title= Physics of fully ionized gas |
|||
|publisher= Interscience tracts of physics and astronomy |
|||
}}</ref> |
|||
A charged particle moving in the direction perpendicular to the magnetic field line is subject to the [[Lorentz force]] which is normal to the plane individuated by the velocity and the magnetic field. This force bends the path of the particle. In general, since particles also have a velocity component along the magnetic field line, the Lorentz force constrains them to bend and move along spirals around the field lines at the [[cyclotron]] frequency. |
|||
If collisions between the particles are very frequent, they are scattered in every direction. This happens in the photosphere, where the plasma carries the magnetic field in its motion. In the corona, on the contrary, the mean free-path of the electrons is of the order of kilometres and even more, so each electron can do a helicoidal motion long before being scattered after a collision. Therefore, the heat transfer is enhanced along the magnetic field lines and inhibited in the perpendicular direction. |
|||
In the direction longitudinal to the magnetic field, the thermal conductivity of the corona is<ref name="Spitzer" /> |
|||
<math display="block"> |
|||
k = 20 \left(\frac{2}{\pi}\right)^{3/2}\frac{\left(k_\text{B} T \right)^{5/2}k_\text{B}}{m_e^{1/2} e^4 \ln \Lambda} \approx \frac{T^{5/2}}{\ln \Lambda} \times 1.8 \times 10^{-10}~ \mathrm{W m^{-1}K^{-1}} |
|||
</math> |
|||
where <math>k_\text{B}</math> is the [[Boltzmann constant]], <math>T</math> is the temperature in kelvin, <math>m_e</math> is the electron mass, <math>e</math> is the electric charge of the electron, |
|||
<math display="block"> \ln \Lambda = \ln \left(12\pi n \lambda_D^3 \right) </math> |
|||
is the Coulomb logarithm, and |
|||
<math display="block">\lambda_D = \sqrt{ \frac{k_\text{B} T }{4 \pi n e^2 }}</math> |
|||
is the [[Debye length]] of the plasma with particle density <math>n</math>. The Coulomb logarithm <math> \ln \Lambda </math> is roughly 20 in the corona, with a mean temperature of 1 MK and a density of 10<sup>15</sup> particles/m<sup>3</sup>, and about 10 in the chromosphere, where the temperature is approximately 10kK and the particle density is of the order of 10<sup>18</sup> particles/m<sup>3</sup>, and in practice it can be assumed constant. |
|||
Thence, if we indicate with <math>q</math> the heat for a volume unit, expressed in J m<sup>−3</sup>, the Fourier equation of heat transfer, to be computed only along the direction <math>x</math> of the field line, becomes |
|||
<math display="block"> \frac{\partial q}{\partial t} = 0.9 \times 10^{-11}~ \frac{\partial^2 T^{7/2}}{\partial x ^2 }.</math> |
|||
Numerical calculations have shown that the thermal conductivity of the corona is comparable to that of copper. |
|||
===Coronal seismology=== |
|||
{{main|Coronal seismology}} |
|||
Coronal seismology is a method of studying the plasma of the solar corona with the use of [[magnetohydrodynamic]] (MHD) waves. MHD studies the [[dynamics (mechanics)|dynamics]] of [[electrical conduction|electrically conducting]] [[fluid]]s — in this case, the fluid is the coronal plasma. Philosophically, coronal seismology is similar to the Earth's [[seismology]], the Sun's [[helioseismology]], and MHD spectroscopy of laboratory plasma devices. In all these approaches, waves of various kinds are used to probe a medium. The potential of coronal seismology in the estimation of the coronal magnetic field, density [[scale height]], [[fine structure]] and heating has been demonstrated by different research groups. |
|||
==Coronal heating problem== |
|||
{{unsolved|physics|Why is the Sun's corona so much hotter than the Sun's surface?}} |
|||
[[File:Van Gogh Sun.ogv|thumb|A new visualisation technique can provide clues to the coronal heating problem.]] |
|||
The coronal heating problem in [[solar physics]] relates to the question of why the temperature of the Sun's corona is millions of kelvins versus the thousands of kelvins of the surface. Several theories have been proposed to explain this phenomenon but it is still challenging to determine which of these is correct.<ref name=":0">{{Cite web|url=http://adsbit.harvard.edu/cgi-bin/nph-iarticle_query?bibcode=2004ESASP.575....2K&db_key=AST&page_ind=0&data_type=GIF&type=SCREEN_VIEW&classic=YES|title=2004ESASP.575....2K Page 2|website=adsbit.harvard.edu|access-date=2019-02-28}}</ref> The problem first emerged when [[Bengt Edlén|Bengt Edlen]] and [[Walter Grotrian]] identified Fe IX and Ca XIV lines in the solar spectrum.<ref name=":1">{{Cite book|title=Physics of the Solar Corona: An Introduction with Problems and Solutions|url=https://archive.org/details/physicssolarcoro00asch_102|url-access=limited|last=Aschwanden|first=Markus|publisher=Springer Science & Business Media|year=2006|isbn=978-3540307655|location=Berlin|pages=[https://archive.org/details/physicssolarcoro00asch_102/page/n373 355]}}</ref> This led to the discovery that the emission lines seen during solar eclipses are not caused by an unknown element called "coronium" but known elements at very high stages of ionization.<ref name=":0" /> The comparison of the coronal and the photospheric temperatures of 6,000K, leads to the question of how the 200 times hotter coronal temperature can be maintained.<ref name=":1" /> The problem is primarily concerned with how the energy is transported up into the corona and then converted into heat within a few solar radii.<ref>{{Cite book|title=Turbulence and Magnetic Fields in Astrophysics|url=https://archive.org/details/turbulencemagnet00falg_199|url-access=limited|last1=Falgarone|first1=Edith|last2=Passot|first2=Thierry|publisher=Springer Science & Business Media|year=2003|isbn=978-3540002741|location=Berlin|pages=[https://archive.org/details/turbulencemagnet00falg_199/page/n33 28]}}</ref> |
|||
The high temperatures require energy to be carried from the solar interior to the corona by non-thermal processes, because the [[second law of thermodynamics]] prevents heat from flowing directly from the solar photosphere (surface), which is at about {{gaps|5|800|K}}, to the much hotter corona at about 1 to 3 [[SI prefix|MK]] (parts of the corona can even reach {{gaps|10|MK}}). |
|||
Between the photosphere and the corona, the thin region through which the temperature increases is known as the [[solar transition region|transition region]]. It ranges from only tens to hundreds of kilometers thick. Energy cannot be transferred from the cooler photosphere to the corona by conventional heat transfer as this would violate the second law of thermodynamics. An analogy of this would be a light bulb raising the temperature of the air surrounding it to something greater than its glass surface. Hence, some other manner of energy transfer must be involved in the heating of the corona. |
|||
The amount of power required to heat the solar corona can easily be calculated as the difference between coronal radiative losses and heating by thermal conduction toward the chromosphere through the transition region. It is about 1 kilowatt for every square meter of surface area on the Sun's chromosphere, or 1/{{gaps|40|000}} of the amount of light energy that escapes the Sun. |
|||
Many coronal heating theories have been proposed,<ref>{{cite book |last = Ulmshneider |first= Peter |title = Heating of Chromospheres and Coronae in ''Space Solar Physics'', Proceedings, Orsay, France |editor = J.C. Vial |editor2 = K. Bocchialini |editor3 = P. Boumier| publisher = Springer | pages = 77–106| year = 1997| isbn= 978-3-540-64307-4}}</ref> but two theories have remained as the most likely candidates: wave heating and [[magnetic reconnection]] (or [[nanoflares]]).<ref>{{cite book |last1=Malara |first1= F. |last2=Velli |first2= M. |title = Observations and Models of Coronal Heating in ''Recent Insights into the Physics of the Sun and Heliosphere: Highlights from SOHO and Other Space Missions'', Proceedings of IAU Symposium 203 |editor = Pål Brekke |editor2 = Bernhard Fleck |editor3 = Joseph B. Gurman| publisher = Astronomical Society of the Pacific | pages = 456–466| year = 2001| isbn= 978-1-58381-069-9}}</ref> Through most of the past 50 years, neither theory has been able to account for the extreme coronal temperatures. |
|||
In 2012, high resolution (<0.2″) [[soft X-ray]] imaging with the [[High Resolution Coronal Imager]] aboard a [[sounding rocket]] revealed tightly wound braids in the corona. It is hypothesized that the reconnection and unravelling of braids can act as primary sources of heating of the active solar corona to temperatures of up to 4 million kelvin. The main heat source in the quiescent corona (about 1.5 million kelvin) is assumed to originate from MHD waves.<ref name=Cirtain2013>{{Cite journal | last1 = Cirtain | first1 = J. W. | last2 = Golub | first2 = L. | last3 = Winebarger | first3 = A. R. | last4 = De Pontieu | first4 = B. | last5 = Kobayashi | first5 = K. | last6 = Moore | first6 = R. L. | last7 = Walsh | first7 = R. W. | last8 = Korreck | first8 = K. E. | last9 = Weber | first9 = M. | doi = 10.1038/nature11772 | last10 = McCauley | first10 = P. | last11 = Title | first11 = A. | last12 = Kuzin | first12 = S. | last13 = Deforest | first13 = C. E. | title = Energy release in the solar corona from spatially resolved magnetic braids | journal = Nature | volume = 493 | issue = 7433 | pages = 501–503 | year = 2013 | pmid = 23344359|bibcode = 2013Natur.493..501C | s2cid = 205232074 }}</ref> |
|||
[[NASA]]'s [[Parker Solar Probe]] is intended to approach the Sun to a distance of approximately 9.5 solar radii to investigate coronal heating and the origin of the solar wind. It was successfully launched on August 12, 2018<ref>{{cite web |url=http://parkersolarprobe.jhuapl.edu/The-Mission/index.php#Journey-to-the-Sun |title= Parker Solar Probe: The Mission|website=parkersolarprobe.jhuapl.edu |archive-url=https://web.archive.org/web/20170822222954/http://parkersolarprobe.jhuapl.edu/The-Mission/index.php |archive-date=2017-08-22}}</ref> and as of fall 2022 has completed the first 13 of more than 20 planned close approaches to the Sun.<ref>{{Cite web|url=https://blogs.nasa.gov/parkersolarprobe/2019/09/03/parker-solar-probe-completes-third-close-approach-of-the-sun/|title=Parker Solar Probe Completes Third Close Approach of the Sun|website=blogs.nasa.gov|date=3 September 2019 |access-date=2019-12-06}}</ref> |
|||
{| class="wikitable" style="margin: 1em auto 1em auto" |
|||
|+'''Competing heating mechanisms''' |
|||
|- |
|||
! colspan="3" |Heating Models |
|||
|- |
|||
! Hydrodynamic |
|||
! colspan="2" |Magnetic |
|||
|- |
|||
| rowspan="2" | |
|||
* No magnetic field |
|||
* Slow rotating stars |
|||
! [[Direct current|DC]] (''reconnection'') |
|||
! [[Alternating current|AC]] (''waves'') |
|||
|- |
|||
| |
|||
* B-field stresses |
|||
* Reconnection events |
|||
* Flares-nanoflares |
|||
* ''Uniform heating rates'' |
|||
| |
|||
* Photospheric foot point ''shuffling'' |
|||
* MHD wave propagation |
|||
* High Alfvén wave flux |
|||
* ''Non-uniform heating rates'' |
|||
|- |
|||
! |
|||
! colspan="2" |Competing theories |
|||
|} |
|||
===Wave heating theory=== |
|||
The wave heating theory, proposed in 1949 by [[Évry Schatzman]], proposes that waves carry energy from the solar interior to the solar chromosphere and corona. The Sun is made of plasma rather than ordinary gas, so it supports several types of waves analogous to [[sound waves]] in air. The most important types of wave are [[magneto-acoustic wave]]s and [[Alfvén wave]]s.<ref>{{cite journal |
|||
| last = Alfvén |
|||
| first = Hannes |
|||
| title = Magneto hydrodynamic waves, and the heating of the solar corona |
|||
| journal = MNRAS |
|||
| volume = 107 |
|||
| issue = 2 |
|||
| pages = 211–219 |
|||
| year = 1947 |
|||
|bibcode = 1947MNRAS.107..211A |
|||
| doi=10.1093/mnras/107.2.211| doi-access = free |
|||
}}</ref> Magneto-acoustic waves are sound waves that have been modified by the presence of a magnetic field, and Alfvén waves are similar to [[ultra low frequency]] [[radio waves]] that have been modified by interaction with [[matter]] in the plasma. Both types of waves can be launched by the turbulence of [[Granule (solar physics)|granulation]] and [[super granulation]] at the solar photosphere, and both types of waves can carry energy for some distance through the solar atmosphere before turning into [[shock waves]] that dissipate their energy as heat. |
|||
One problem with wave heating is delivery of the heat to the appropriate place. Magneto-acoustic waves cannot carry sufficient energy upward through the chromosphere to the corona, both because of the low pressure present in the chromosphere and because they tend to be [[reflection (physics)|reflected]] back to the photosphere. Alfvén waves can carry enough energy, but do not dissipate that energy rapidly enough once they enter the corona. Waves in plasmas are notoriously difficult to understand and describe analytically, but computer simulations, carried out by Thomas Bogdan and colleagues in 2003, seem to show that Alfvén waves can transmute into other wave modes at the base of the corona, providing a pathway that can carry large amounts of energy from the photosphere through the chromosphere and transition region and finally into the corona where it dissipates it as heat. |
|||
Another problem with wave heating has been the complete absence, until the late 1990s, of any direct evidence of waves propagating through the solar corona. The first direct observation of waves propagating into and through the solar corona was made in 1997 with the [[Solar and Heliospheric Observatory]] space-borne solar observatory, the first platform capable of observing the Sun in the [[extreme ultraviolet]] (EUV) for long periods of time with stable [[Photometry (astronomy)|photometry]]. Those were magneto-acoustic waves with a frequency of about 1 [[hertz|millihertz]] (mHz, corresponding to a {{gaps|1|000|second}} wave period), that carry only about 10% of the energy required to heat the corona. Many observations exist of localized wave phenomena, such as Alfvén waves launched by solar flares, but those events are transient and cannot explain the uniform coronal heat. |
|||
It is not yet known exactly how much wave energy is available to heat the corona. Results published in 2004 using data from the [[TRACE]] spacecraft seem to indicate that there are waves in the solar atmosphere at frequencies as high as {{gaps|100|mHz}} (10 second period). Measurements of the temperature of different [[ions]] in the solar wind with the UVCS instrument aboard [[Solar and Heliospheric Observatory|SOHO]] give strong indirect evidence that there are waves at frequencies as high as {{gaps|200|Hz}}, well into the range of human hearing. These waves are very difficult to detect under normal circumstances, but evidence collected during solar eclipses by teams from [[Williams College]] suggest the presences of such waves in the 1–{{gaps|10|Hz}} range. |
|||
Recently, Alfvénic motions have been found in the lower solar atmosphere<ref>{{cite web |url=http://www.science20.com/news_releases/alfven_waves_our_sun_doing_magnetic_twist |title=Alfven Waves – Our Sun Is Doing The Magnetic Twist |publisher=read on Jan 6 2011 |url-status=live |archive-url=https://web.archive.org/web/20110723053337/http://www.science20.com/news_releases/alfven_waves_our_sun_doing_magnetic_twist |archive-date=2011-07-23 }}</ref><ref>{{cite journal | doi = 10.1126/science.1168680 | last1 = Jess | first1 = DB | last2 = Mathioudakis | first2 = M | last3 = Erdélyi | first3 = R | last4 = Crockett | first4 = PJ | last5 = Keenan | first5 = FP | last6 = Christian | first6 = DJ | title = Alfvén Waves in the Lower Solar Atmosphere | journal = Science | volume = 323| issue = 5921 | pages = 1582–1585 | year = 2009 | pmid = 19299614|bibcode = 2009Sci...323.1582J |arxiv = 0903.3546 | hdl = 10211.3/172550 | s2cid = 14522616 }}</ref> and also in the quiet Sun, in coronal holes and in active regions using observations with AIA on board the [[Solar Dynamics Observatory]].<ref>{{cite journal|last1=McIntosh |first1= S. W. |last2=de Pontieu |first2= B. |last3=Carlsson |first3= M. |last4=Hansteen |first4= V. H. |author5=The Sdo |author6=Aia Mission Team | title = Ubiquitous Alfvenic Motions in Quiet Sun, Coronal Hole and Active Region Corona |
|||
| journal = American Geophysical Union, Fall Meeting |
|||
| volume = abstract #SH14A-01 |
|||
| year = 2010 |
|||
|pages=SH14A–01 |bibcode=2010AGUFMSH14A..01M }}</ref> |
|||
These Alfvénic oscillations have significant power, and seem to be connected to the chromospheric Alfvénic oscillations previously reported with the [[Hinode (satellite)|Hinode]] spacecraft.<ref>{{cite web |
|||
|url = http://www.space.com/scienceastronomy/080122-st-sunshine-hinode.html |
|||
|title = Sun's Magnetic Secret Revealed |
|||
|date = 22 January 2008 |
|||
|publisher = read on Jan 6 2011 |
|||
|url-status = live |
|||
|archive-url = https://web.archive.org/web/20101224061437/http://www.space.com/scienceastronomy/080122-st-sunshine-hinode.html |
|||
|archive-date = 2010-12-24 |
|||
}}</ref> |
|||
Solar wind observations with the [[Wind (spacecraft)|''Wind'']] spacecraft have recently shown evidence to support theories of Alfvén-cyclotron dissipation, leading to local ion heating.<ref>{{cite journal|last=Kasper|first=J.C.|title=Hot Solar-Wind Helium: Direct Evidence for Local Heating by Alfven-Cyclotron Dissipation|journal=Phys. Rev. Lett.|date=December 2008|volume=101|pmid=19113766|issue=26|page=261103|doi=10.1103/PhysRevLett.101.261103|bibcode=2008PhRvL.101z1103K|display-authors=etal}}</ref> |
|||
===Magnetic reconnection theory=== |
|||
{{main|Magnetic reconnection}} |
|||
[[File:Arcing Active Region.jpg|thumb|Arcing active region by [[Solar Dynamics Observatory]]]] |
|||
The magnetic reconnection theory relies on the solar magnetic field to induce electric currents in the solar corona.<ref>{{cite book |
|||
| last = Priest |
|||
| first = Eric |
|||
| title = Solar Magneto-hydrodynamics |
|||
| publisher = D.Reidel Publishing Company, Dordrecht, Holland |
|||
| year = 1982 |
|||
| isbn = 978-90-277-1833-4 |
|||
}}</ref> The currents then collapse suddenly, releasing energy as heat and wave energy in the corona. This process is called "reconnection" because of the peculiar way that magnetic fields behave in plasma (or any electrically conductive fluid such as [[Mercury (element)|mercury]] or [[seawater]]). In a plasma, [[magnetic field lines]] are normally tied to individual pieces of matter, so that the [[topology]] of the magnetic field remains the same: if a particular north and south [[Poles of astronomical bodies#Magnetic poles|magnetic pole]] are connected by a single field line, then even if the plasma is stirred or if the magnets are moved around, that field line will continue to connect those particular poles. The connection is maintained by electric currents that are induced in the plasma. Under certain conditions, the electric currents can collapse, allowing the magnetic field to "reconnect" to other magnetic poles and release heat and wave energy in the process. |
|||
Magnetic reconnection is hypothesized to be the mechanism behind solar flares, the largest explosions in the Solar System. Furthermore, the surface of the Sun is covered with millions of small magnetized regions 50–{{gaps|1|000|km}} across. These small magnetic poles are buffeted and churned by the constant granulation. The magnetic field in the solar corona must undergo nearly constant reconnection to match the motion of this "magnetic carpet", so the energy released by the reconnection is a natural candidate for the coronal heat, perhaps as a series of "microflares" that individually provide very little energy but together account for the required energy. |
|||
The idea that nanoflares might heat the corona was proposed by Eugene Parker in the 1980s but is still controversial. In particular, [[ultraviolet]] telescopes such as [[TRACE]] and [[Solar and Heliospheric Observatory|SOHO]]/EIT can observe individual micro-flares as small brightenings in extreme ultraviolet light,<ref>{{cite journal |
|||
| doi = 10.1051/0004-6361:20020151 |
|||
| last1 = Patsourakos |
|||
| first1 = S. |
|||
| last2 = Vial |
|||
| first2 = J.-C. |
|||
| title = Intermittent behavior in the transition region and the low corona of the quiet Sun |
|||
| journal = Astronomy and Astrophysics |
|||
| volume = 385 |
|||
| issue = 3 |
|||
| pages = 1073–1077 |
|||
| year = 2002 |
|||
| bibcode=2002A&A...385.1073P |
|||
| doi-access = free |
|||
}}</ref> but there seem to be too few of these small events to account for the energy released into the corona. The additional energy not accounted for could be made up by wave energy, or by gradual magnetic reconnection that releases energy more smoothly than micro-flares and therefore doesn't appear well in the TRACE data. Variations on the micro-flare hypothesis use other mechanisms to stress the magnetic field or to release the energy, and are a subject of active research in 2005. |
|||
===Spicules (type II)=== |
|||
For decades, researchers believed spicules could send heat into the corona. However, following observational research in the 1980s, it was found that spicule plasma did not reach coronal temperatures, and so the theory was discounted. |
|||
As per studies performed in 2010 at the ''National Center for Atmospheric Research'' in [[Colorado]], in collaboration with the ''Lockheed Martin's Solar and Astrophysics Laboratory'' (LMSAL) and the ''Institute of Theoretical Astrophysics'' of the [[University of Oslo]], a new class of spicules (TYPE II) discovered in 2007, which travel faster (up to 100 km/s) and have shorter lifespans, can account for the problem.<ref>{{cite web |url=http://www.rediff.com/news/slide-show/slide-show-1-mystery-of-suns-hot-outer-atmosphere-solved/20110107.htm |title=Mystery of Sun's hot outer atmosphere 'solved' – Rediff.com News |work=Rediff.com |date=2011-01-07 |access-date=2012-05-21 |url-status=live |archive-url=https://web.archive.org/web/20120415111049/http://www.rediff.com/news/slide-show/slide-show-1-mystery-of-suns-hot-outer-atmosphere-solved/20110107.htm |archive-date=2012-04-15 }}</ref> These jets insert heated plasma into the Sun's outer atmosphere. |
|||
Thus, a much greater understanding of the corona and improvement in the knowledge of the Sun's subtle influence on the Earth's upper atmosphere can be expected henceforth. The Atmospheric Imaging Assembly on NASA's recently launched Solar Dynamics Observatory and NASA's Focal Plane Package for the Solar Optical Telescope on the Japanese Hinode satellite which was used to test this hypothesis. The high spatial and temporal resolutions of the newer instruments reveal this coronal mass supply. |
|||
These observations reveal a one-to-one connection between plasma that is heated to millions of degrees and the spicules that insert this plasma into the corona.<ref>{{cite journal | doi = 10.1126/science.1197738 | last1 = De Pontieu | first1 = B | last2 = McIntosh | first2 = SW | last3 = Carlsson | first3 = M | last4 = Hansteen | first4 = VH | last5 = Tarbell | first5 = TD | last6 = Boerner | first6 = P | last7 = Martinez-Sykora | first7 = J | last8 = Schrijver | first8 = CJ | last9 = Title | first9 = AM | title = The Origins of Hot Plasma in the Solar Corona | journal = Science | volume = 331| issue = 6013 | pages = 55–58| year = 2011 | pmid = 21212351|bibcode = 2011Sci...331...55D | s2cid = 42068767 }}</ref> |
|||
--> |
|||
== Vegeu també == |
== Vegeu també == |
Revisió del 22:06, 29 ago 2023
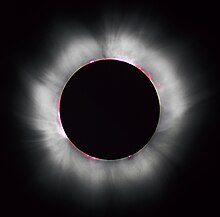
La corona solar és la part exterior de l'atmosfera del Sol. Està situada entre la regió de transició i l'heliosfera. Fa més d'un milió de quilòmetres i pot observar-se durant els eclipsis solars o utilitzant un dispositiu capaç d'ocultar la llum del Sol, denominat coronògraf. La densitat de la corona solar és un bilió de vegades inferior a la de l'atmosfera terrestre i la seua temperatura aconseguix els dos milions de graus (mentre que la fotosfera té una temperatura aproximada de 6.000 °C).
Descripció
Es presenta com una o diverses sèries, normalment no més de tres, d'anells acolorits centrats sobre el Sol o la Lluna i de radi relativament petit. En cada sèrie d'anells, l'interior és de color violeta o blau i l'exterior vermell. Poden arribar a distingir-s'hi, de vegades, tots els colors de l'espectre. Generalment, aquests colors són més fàcilment visibles en les corones lunars. Molt sovint, se'n redueix a una primera sèrie pròxima a l'astre i de menys de 5º de radi: l'aurèola és de color blanc blavenc amb vores rogenques.[1]
El fenomen és causat per la difracció o la dispersió de la llum en núvols prims o en la boira. Els raigs lluminosos de l'astre es dispersen en topar amb les gotes d'aigua i surten desviats. L'angle de desviació n'augmenta quan disminueix el diàmetre de la gota d'aigua. Si el diàmetre de les gotes és força homogeni, els contorns dels anells seran molt més nítids. Els núvols que generen la corona estan formats per gotes d'aigua, no per cristalls de glaç. En la major part dels casos, es tracta d'altocúmuls, tot i que també se'n poden observar en quedar el disc solar matisat per l'obstacle d'un altoestrat, un estrat o molt rarament, d'un cirrocúmul.[1]
Per a observar la corona solar, convé no confondre-la amb l'halo; una corona mai no arriba a ser ni la meitat de gran que l'halo. La corona sovint és difusa, el contorn de l'halo sempre està ben definit. Les corones són de grandària variable. Els halos són sempre iguals. Popularment, es diu que la corona, en deixar veure menys llum solar, es pot mirar directament sense protecció als ulls, i amb els halos això no és possible. No s'aconsella refiar-se d'aquest sistema d'identificació.[1]
Estudi de la corona solar
- Durant un eclipsi, el 1870, Charles Young, observant l'espectre de llum de la corona, va identificar-hi un traç verd l'origen del qual no va poder ser explicat. Entre les hipòtesis que van circular en l'època, es va parlar d'un suposat element químic desconegut que no estaria disponible a la Terra.
- Fins al 1930, l'única manera d'observar la corona era durant els eclipsis totals de Sol.
- Gràcies a la invenció, el 1930, d'un enginyós dispositiu per a produir eclipsis artificials, els anomenats coronògrafs, es va poder estudiar de manera més accessible el fenomen de la corona solar.
- El 1940, Edlen i de Grotrian van demostrar que les ratlles verdes no eren produïdes per l'espectre de materials desconeguts, sinó per àtoms altament ionitzats d'elements disponibles a la Terra, com el ferro.
Característiques físiques

La corona solar és molt més calenta (en un factor de 150 a 450) que la superfície visible del Sol: la temperatura de la corona és d'1 a 3 milions de kelvin en comparació amb la temperatura mitjana de la fotosfera, al voltant dels 5800kelvin. La corona és 10−12 vegades més densa que la fotosfera i, per tant, produeix aproximadament una mil·lèsima part de llum visible. La corona està separada de la fotosfera per la relativament poc profunda cromosfera. El mecanisme exacte pel qual s'escalfa la corona encara és objecte de cert debat, però les possibilitats probables inclouen la inducció pel camp magnètic del Sol i les ones magnetohidrodinàmiques des de sota. Les parts exteriors de la corona solar estan sent transportades constantment a causa del flux magnètic obert i, per tant, genera el vent solar.
La corona no sempre es distribueix uniformement per la superfície solar. Durant els períodes de tranquil·litat, la corona està més o menys confinada a les regions equatorials, amb forats coronals que cobreixen les regions polars. Tanmateix, durant els períodes actius solars, la corona es distribueix uniformement per les regions equatorials i polars, tot i que és més prominent a les zones amb activitat de taques solars. El cicle solar abasta aproximadament 11 anys, des del mínim solar fins al mínim següent. Com que el camp magnètic solar s'enrotlla contínuament a causa de la rotació més ràpida de la massa a l'equador del Sol (rotació diferencial), L'activitat de les taques solars serà més pronunciada al màxim solar on el camp magnètic està més retorçat. Associades a les taques solars hi ha bucles coronals , bucles de flux magnètic , surgència de l'interior solar. El flux magnètic allunya la fotosfera més calenta a un costat, exposant el plasma més fresc a sota; per tant, així es crea les taques solars relativament fosques.
Com que la corona ha estat fotografiada a alta resolució en el rang de raigs X de l'espectre pel satèl·lit Skylab l'any 1973, i després pel Yohkoh i els altres instruments espacials següents, s'ha vist que l'estructura de la corona és força variada. i complexa: s'han classificat immediatament diferents zones al disc coronal.[2][3][4] Els astrònoms solen distingir diverses regions,[5] com es descriu a continuació.
Regions actives
Les regions actives són conjunts d'estructures de rínxol que connecten punts de polaritat magnètica oposada a la fotosfera, els anomenats bucles coronals. Generalment es distribueixen en dues zones d'activitat, que són paral·leles a l'equador solar. La temperatura mitjana és d'entre dos i quatre milions de kelvin, mentre que la densitat va de 109 a 1010 partícules per cm3.

Les regions actives impliquen tots els fenòmens directament relacionats amb el camp magnètic, que es produeixen a diferents altures sobre la superfície solar:[5] les taques solars i les fàcules es produeixen a la fotosfera; espícules, filaments Hα i platges a la cromosfera; prominències a la cromosfera i la regió de transició; i les erupcions i les ejeccions de massa coronal es produeixen a la corona i la cromosfera. Si les bengales són molt violentes, també poden pertorbar la fotosfera i generar una ona Moreton. Per contra, les prominències quiescents són estructures grans, fresques i denses que s'observen com a cintes Hα fosques, "com una serp" (que apareixen com a filaments) al disc solar. La seva temperatura és d'uns 5000–8000K, per la qual cosa se solen considerar com a característiques cromosfèriques.
El 2013, les imatges del High Resolution Coronal Imager van revelar "trences magnètiques" mai vistes de plasma dins de les capes exteriors d'aquestes regions actives.[6]
Rínxol coronal

Els rínxols coronals són les estructures bàsiques de la corona solar magnètica. Aquests bucles són els cosins de flux magnètic tancat del flux magnètic obert que es pot trobar als forats coronals i al vent solar. Bucles de flux magnètic surten del cos solar i s'omplen de plasma solar calent.[7] A causa de l'augment de l'activitat magnètica en aquestes regions de bucle coronal, els bucles coronals sovint poden ser el precursor de les erupcions solars i les ejeccions de massa coronal.
El plasma solar que alimenta aquestes estructures s'escalfa des de menys de 6000K fins a més de 106 K des de la fotosfera, a través de la regió de transició i cap a la corona. Sovint, el plasma solar omplirà aquests rínxols d'un punt i drenarà a un altre, anomenats punts de peu (flux de sifó a causa d'una diferència de pressió,[8] o flux asimètric a causa d'algun altre conductor).
Quan el plasma puja des dels punts del peu cap a la part superior del rínxol, com passa sempre durant la fase inicial d'una erupció compacta, es defineix com a evaporació cromosfèrica. Quan el plasma se refreda ràpidament i cau cap a la fotosfera, s'anomena condensació cromosfèrica. També pot haver-hi un flux simètric des dels dos punts del peu del rínxol, provocant una acumulació de massa a l'estructura del rínxol. El plasma es pot refredar ràpidament en aquesta regió (per a una inestabilitat tèrmica), els seus filaments foscos són evidents contra el disc solar o les prominències de l'extremitat del Sol.
Els rinxols coronals poden tenir una vida útil de l'ordre de segons (en el cas dels esdeveniments d'espurneig), minuts, hores o dies. Quan hi ha un equilibri en les fonts i embornals d'energia de bucle, els bucles coronals poden durar llargs períodes de temps i es coneixen com a rinxols coronals estat estacionari o quiescent. (exemple).
Els rínxols coronals són molt importants per a la nostra comprensió del problema de l'escalfament coronal actual. Els rínxols coronals són fonts de plasma molt radiants i, per tant, són fàcils d'observar amb instruments com ara TRACE. Es manté una explicació del problema de l'escalfament coronal, ja que aquestes estructures s'estan observant de forma remota, on hi ha moltes ambigüitats (és a dir, contribucions de radiació al llarg de la propagació d'abast visual). Les mesures In-situ són necessàries abans que es pugui determinar una resposta definitiva, però a causa de les altes temperatures del plasma a la corona, les mesures in-situ són, actualment, impossibles. La propera missió de la Parker Solar Probe de la NASA s'aproparà al Sol molt de prop, on permetrà observacions més directes.
Estructures a gran escala
Les estructures a gran escala són arcs molt llargs que poden cobrir més d'una quarta part del disc solar però contenen plasma menys dens que en els rínxols coronals de les regions actives.
Es van detectar per primera vegada el 8 de juny de 1968, l'observació d'una bengala durant un vol de coet.[9]
L'estructura a gran escala de la corona canvia al llarg del cicle solar d'11 anys i esdevé particularment simple durant el període mínim, quan el camp magnètic del Sol és gairebé similar a una configuració dipolar (a més d'un component quadrupolar).
Interconnexions de regions actives
Les interconnexions de regions actives són arcs que connecten zones de camp magnètic oposat, de regions actives diferents. Sovint es veuen variacions significatives d'aquestes estructures després d'una erupció.[10]
Algunes altres característiques d'aquest tipus són les plomalls coronals: grans estructures coronals semblants a un casquet amb cims llargs i punxeguts que solen sobreposar-se a taques solars i regions actives. Els plomalls coronals es consideren fonts del vent solar lent.[10]
Cavitats de filaments

Les cavitats dels filaments són zones que semblen fosques als raigs X i es troben per sobre de les regions on s'observen filaments Hα a la cromosfera. Es van observar per primera vegada en els dos vols de coets de 1970 que també van detectar forats coronals.[9]
Les cavitats dels filaments són núvols de plasma més freds suspesos per sobre de la superfície del Sol per forces magnètiques. Les regions de camp magnètic intens semblen fosques a les imatges perquè estan buides de plasma calent. De fet, la suma de la pressió magnètica i la pressió del plasma ha de ser constant a tot arreu de l'heliosfera per tal de tenir una configuració d'equilibri: on el camp magnètic és més alt, el plasma ha de ser més fred o menys dens. La pressió del plasma es pot calcular mitjançant l'equació d'estat d'un gas perfecte: , on és la densitat del nombre de partícules, la constant de Boltzmann i la temperatura del plasma. A partir de l'equació és evident que la pressió del plasma baixa quan la temperatura del plasma disminueix respecte a les regions circumdants o quan es buida la zona de camp magnètic intens. El mateix efecte físic fa que les taques solars aparentment fosques a la fotosfera.
Punts brillants
Els punts brillants són petites regions actives que es troben al disc solar. Els punts brillants de raigs X es van detectar per primera vegada el 8 d'abril de 1969, durant el vol d'un coet.[9]
La fracció de la superfície solar coberta per punts brillants varia amb el cicle solar. Estan associats a petites regions bipolars del camp magnètic. La seva temperatura mitjana oscil·la entre 1,1 MK i 3,4 MK. Les variacions de temperatura sovint estan correlacionades amb els canvis en l'emissió de raigs X.[11]
Forat coronal
Els forats coronals són regions unipolars que apareixen foscos als raigs X ja que no emeten molta radiació.[12] Són zones àmplies solars on el camp magnètic és unipolar i s'obre cap a l'espai interplanetari. El vent solar d'alta velocitat sorgeix principalment d'aquestes regions.
A les imatges ultraviolades dels forats coronals, sovint es veuen algunes estructures petites, similars a bombolles allargades, suspeses al vent solar. Aquests són els plomalls coronals. Més precisament, són serpentines llargues i primes que es projecten cap a l'exterior des dels pols nord i sud del Sol.[13]
El Sol tranquil
Les regions solars que no formen part de les regions actives i els forats coronals s'identifiquen comunament com el Sol tranquil.
La regió equatorial té una velocitat de rotació més ràpida que les zones polars. El resultat de la rotació diferencial del Sol és que les regions actives sorgeixen sempre en dues bandes paral·leles a l'equador i la seva extensió augmenta durant els períodes de màxim del cicle solar, mentre que gairebé desapareixen durant cada mínim. Per tant, el Sol tranquil sempre coincideix amb la zona equatorial i la seva superfície és menys activa durant el màxim del cicle solar. Aproximant-se al mínim del cicle solar (també anomenat cicle de la papallona), l'extensió del Sol tranquil augmenta fins a cobrir tota la superfície del disc excloent alguns punts brillants de l'hemisferi i els pols, on hi ha forats coronals.
Punt d'Alfvén
El punt d'Alfvén és el límit que separa la corona del vent solar definit com on la velocitat d'Alfvén del plasma coronal i la velocitat del vent solar a gran escala són iguals.[14][15]
Els investigadors no estaven segurs exactament on es trobava la superfície crítica solar d'Alfvén. A partir d'imatges remotes de la corona, les estimacions l'havien situat entre 10 i 20 radis solars de la superfície del Sol. El 28 d'abril de 2021, durant el seu vuitè sobrevol del Sol, la Parker Solar Probe de la NASA es va trobar amb les condicions magnètiques i de partícules específiques a 18,8 radis solars que indicaven que va penetrar al punt d'Alfvén.[16]
Variabilitat de la corona
Un retrat, tan diversificat com el ja assenyalat per als trets coronals, es destaca per l'anàlisi de la dinàmica de les principals estructures de la corona, que evolucionen en temps diferencials. Estudiar la variabilitat coronal en la seva complexitat no és fàcil perquè els temps d'evolució de les diferents estructures poden variar considerablement: des de segons fins a diversos mesos. Les mides típiques de les regions on es produeixen esdeveniments coronals varien de la mateixa manera, tal com es mostra a la taula següent.
Esdeveniment coronal | Escala de temps típica | Escala de longitud típica (Mm) |
---|---|---|
Erupció de la regió activa | 10 to 10000seconds | 10–100 |
Punt brillant de raigs X | minuts | 1–10 |
Transitòria en estructures de gran escala | de minuts a hores | ~100 |
Transitori en arcs d'interconnexió | de minuts a hores | ~100 |
Sol tranquil | d'hores a mesos | 100–1000 |
Foral coronal | diverses rotacions | 100–1000 |
Erupcions

Les erupcions tenen lloc a les regions actives i es caracteritzen per un augment sobtat del flux radiatiu emès des de petites regions de la corona. Són fenòmens molt complexos, visibles a diferents longituds d'ona; impliquen diverses zones de l'atmosfera solar i molts efectes físics, tèrmics i no tèrmics, i de vegades àmplies reconnexions de les línies de camp magnètic amb expulsió de material.
Les erupcions són fenòmens impulsius, de durada mitjana de 15 minuts, i els esdeveniments més energètics poden durar diverses hores. Les erupcions produeixen un augment elevat i ràpid de la densitat i la temperatura.
Poques vegades s'observa una emissió en llum blanca: normalment, les erupcions només es veuen a longituds d'ona ultravioleta extremes i als raigs X, típics de l'emissió cromosfèrica i coronal.
A la corona, la morfologia de les erupcions es descriu mitjançant observacions en raigs ultraviolats, raigs X suaus i durs i en longituds d'ona Hα, i és molt complexa. Tot i així, es poden distingir dos tipus d'estructures bàsiques: [17]
- Erupcions compactes, quan cadascun dels dos arcs on està succeint l'esdeveniment manté la seva morfologia: només s'observa un augment de l'emissió sense variacions estructurals importants. L'energia emesa és de l'ordre de 1022 – 1023 J.
- Erupcions de llarga durada, associades a erupcions de prominències, transitoris en llum blanca i erupcions de dues cintes:[18] en aquest cas els rinxols magnètics canvien la seva configuració durant l'esdeveniment. Les energies emeses durant aquestes erupcions són d'una proporció tan gran que poden arribar als 1025 J.

Pel que fa a la dinàmica temporal, generalment es distingeixen tres fases diferents, la durada de les quals no és comparable. La durada d'aquests períodes depèn del rang de longituds d'ona utilitzats per observar l'esdeveniment:
- Una fase impulsiva inicial, la durada de la qual és de l'ordre de minuts, sovint s'observen fortes emissions d'energia fins i tot en les microones, longituds d'ona EUV i en les freqüències dures de raigs X.
- Una fase màxima
- Una fase de decadència, que pot durar diverses hores.
De vegades també es pot observar una fase que precedeix l'erupció, normalment anomenada fase "preerupció".
Ejeccions de massa coronal
Sovint, acompanyades de grans erupcions solars i prominències, hi ha ejeccions de massa coronal. Es tracta d'emissions enormes de material coronal i camp magnètic que viatgen cap a l'exterior des del Sol a més d'un milió de quilòmetres per hora, que contenen aproximadament 10 vegades l'energia de la erupció solar o la prominència que les acompanya. Algunes ejeccions de massa coronal més grans poden propulsar centenars de milions de tones de material a l'espai interplanetari a aproximadament 1,5 milions de quilòmetres per hora.
Vegeu també
Referències
- ↑ 1,0 1,1 1,2 «Corona solar i lunar». Meteocat. Gencat. [Consulta: 24 febrer 2015].
- ↑ Vaiana, G. S.; Krieger, A. S.; Timothy, A. F. «Identification and analysis of structures in the corona from X-ray photography» (en anglès). Solar Physics, 32, 1, 1973, pàg. 81–116. Bibcode: 1973SoPh...32...81V. DOI: 10.1007/BF00152731.
- ↑ Vaiana, G.S.; Tucker, W.H.. «Solar X-Ray Emission». A: R. Giacconi. X-Ray Astronomy (en anglès), 1974, p. 169.
- ↑ Vaiana, G S; Rosner, R. «Recent advances in Coronae Physics» (en anglès). Annu. Rev. Astron. Astrophys., 16, 1978, pàg. 393–428. Bibcode: 1978ARA&A..16..393V. DOI: 10.1146/annurev.aa.16.090178.002141.
- ↑ 5,0 5,1 Gibson, E. G.. National Aeronautics and Space Administration, Washington, D.C.. The Quiet Sun (en anglès), 1973.
- ↑ «How NASA Revealed Sun's Hottest Secret in 5-Minute Spaceflight». Space.com, 23-01-2013. Arxivat de l'original el 24 de gener de 2013.
- ↑ Katsukawa, Yukio; Tsuneta, Saku «Magnetic Properties at Footpoints of Hot and Cool Loops» (en anglès). The Astrophysical Journal, 621, 1, 2005, pàg. 498–511. Bibcode: 2005ApJ...621..498K. DOI: 10.1086/427488.
- ↑ Betta, Rita; Orlando, Salvatore; Peres, Giovanni; Serio, Salvatore «On the Stability of Siphon Flows in Coronal Loops» (en anglès). Space Science Reviews, 87, 1999, pàg. 133–136. Bibcode: 1999SSRv...87..133B. DOI: 10.1023/A:1005182503751.
- ↑ 9,0 9,1 9,2 Giacconi, Riccardo. J. F. Linsky i S. Serio. G.S. Vaiana memorial lecture in Proceedinds of Physics of Solar and Stellar Coronae: G.S. Vaiana Memorial Symposium (en anglès). Kluwer Academic Publishers-Printed in the Netherlands, 1992, p. 3–19. ISBN 978-0-7923-2346-4.
- ↑ 10,0 10,1 Ofman, Leon «Source regions of the slow solar wind in coronal streamers» (en anglès). Geophysical Research Letters, 27, 18, 2000, pàg. 2885–2888. Bibcode: 2000GeoRL..27.2885O. DOI: 10.1029/2000GL000097.
- ↑ Kariyappa, R.; Deluca, E. E.; Saar, S. H.; Golub, L.; Damé, L.; Pevtsov, A. A.; Varghese, B. A. «Temperature variability in X-ray bright points observed with Hinode/XRT» (en anglès). Astronomy & Astrophysics, 526, 2011, pàg. A78. Bibcode: 2011A&A...526A..78K. DOI: 10.1051/0004-6361/201014878.
- ↑ Ito, Hiroaki; Tsuneta, Saku; Shiota, Daikou; Tokumaru, Munetoshi; Fujiki, Ken'Ichi «Is the Polar Region Different from the Quiet Region of the Sun?» (en anglès). The Astrophysical Journal, 719, 1, 2010, pàg. 131–142. arXiv: 1005.3667. Bibcode: 2010ApJ...719..131I. DOI: 10.1088/0004-637X/719/1/131.
- ↑ Del Zanna, G.; Bromage, B. J. I.; Mason, H. E. «Spectroscopic characteristics of polar plumes» (en anglès). Astronomy & Astrophysics, 398, 2, 2003, pàg. 743–761. Bibcode: 2003A&A...398..743D. DOI: 10.1051/0004-6361:20021628.
- ↑ Adhikari, L.; Zank, G. P.; Zhao, L.-L. «Does Turbulence Turn off at the Alfvén Critical Surface?» (en anglès). The Astrophysical Journal, 876, 30-04-2019, pàg. 26. Bibcode: 2019ApJ...876...26A. DOI: 10.3847/1538-4357/ab141c.
- ↑ DeForest, C. E.; Howard, T. A.; McComas, D. J. «Inbound waves in the solar corona: a direct indicator of Alfvén Surface location» (en anglès). The Astrophysical Journal, 787, 2, 12-05-2014, pàg. 124. arXiv: 1404.3235. Bibcode: 2014ApJ...787..124D. DOI: 10.1088/0004-637X/787/2/124.
- ↑
Aquest article incorpora text d'un treball de contingut lliure. Hatfield, Miles. «NASA Enters the Solar Atmosphere for the First Time» (en anglès). NASA, 13-12-2021.{{#ifeq: | | {{#if:|}} | }}
Per obtenir informació sobre com afegir text amb llicència lliure als articles de la Viquipèdia, consulteu els termes d'us.
- ↑ Pallavicini, R.; Serio, S.; Vaiana, G. S. «A survey of soft X-ray limb flare images – The relation between their structure in the corona and other physical parameters» (en anglès). The Astrophysical Journal, 216, 1977, pàg. 108. Bibcode: 1977ApJ...216..108P. DOI: 10.1086/155452.
- ↑ Golub, L.; Herant, M.; Kalata, K.; Lovas, I.; Nystrom, G.; Pardo, F.; Spiller, E.; Wilczynski, J. «Sub-arcsecond observations of the solar X-ray corona» (en anglès). Nature, 344, 6269, 1990, pàg. 842–844. Bibcode: 1990Natur.344..842G. DOI: 10.1038/344842a0.